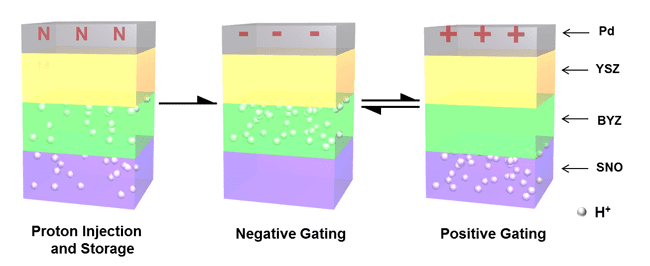
NEW TRANSISTOR ACHIEVES ‘COLOSSAL’ SWITCHABLE RESISTANCE USING QUANTUM MATERIALS AND PHYSICS DEVELOPED IN A FUEL CELL LAB
Silicon has few serious competitors as the material of choice in the electronics industry. Yet transistors, the switchable valves that control the flow of electrons in a circuit, cannot simply keep shrinking to meet the needs of powerful, compact devices; physical limitations like energy consumption and heat dissipation are too significant.
Now, using a quantum material called a correlated oxide, Harvard researchers have achieved a reversible change in electrical resistance of eight orders of magnitude, a result the researchers are calling “colossal.” In short, they have engineered this material to perform comparably with the best silicon switches.
The finding arose in what may seem an unlikely spot: a laboratory usually devoted to studying fuel cells—the kind that run on methane or hydrogen—led by Shriram Ramanathan, Associate Professor of Materials Science at the Harvard School of Engineering and Applied Sciences (SEAS). The researchers’ familiarity with thin films and ionic transport enabled them to exploit chemistry, rather than temperature, to achieve the dramatic result.
Because the correlated oxides can function equally well at room temperature or a few hundred degrees above it, it would be easy to integrate them into existing electronic devices and fabrication methods. The discovery, published in Nature Communications, therefore firmly establishes correlated oxides as promising semiconductors for future three-dimensional integrated circuits as well as for adaptive, tunable photonic devices.
Challenging silicon
Although electronics manufacturers continue to pack greater speed and functionality into smaller packages, the performance of silicon-based components will soon hit a wall.
“Traditional silicon transistors have fundamental scaling limitations,” says Ramanathan. “If you shrink them beyond a certain minimum feature size, they don’t quite behave as they should.”
Yet silicon transistors are hard to beat, with an on/off ratio of at least 104 required for practical use. “It’s a pretty high bar to cross,” Ramanathan explains, adding that until now, experiments using correlated oxides have produced changes of only about a factor of 10, or 100 at most, near room temperature. But Ramanathan and his team have crafted a new transistor, made primarily of an oxide called samarium nickelate, that in practical operation achieves an on/off ratio of greater than 105—that is, comparable to state-of-the-art silicon transistors.
In future work the researchers will investigate the device’s switching dynamics and power dissipation; meanwhile, this advance represents an important proof of concept.
“Our orbital transistor could really push the frontiers of this field and say, you know what? This is a material that can challenge silicon,” Ramanathan says.
The Latest on: Transistor
[google_news title=”” keyword=”Transistor” num_posts=”10″ blurb_length=”0″ show_thumb=”left”]
via Google News
The Latest on: Transistor
- Your complete guide to Offaly’s Esker Arts Centre’s summer line-up of entertainmenton May 2, 2024 at 8:02 am
Radio Luxembourg on transistor radios, and free love remembered with playful precision. Brian Leyden’s books include the bestselling memoir The Home Place and his new novel Love These Days. For three ...
- Third Point - Taiwan Semiconductor: The 'Toll Road' For AI Computeon May 2, 2024 at 7:30 am
We view TSMC as the “toll road” of the semiconductor industry, particularly for AI compute. Click here for our brief investment thesis on TMS stock.
- Make all matriarchs feel loved with the NEXT Mother’s Day Gift Guideon May 2, 2024 at 6:51 am
Moms, aunts, stepmoms, grandmas — we love them all. We went in search of gifts that share local flair for our Mother's Day Gift Guide.
- Research demonstrates high qubit control fidelity and uniformity in single-electron controlon May 2, 2024 at 6:23 am
The journal Nature has published a research paper, "Probing single electrons across 300-mm spin qubit wafers," demonstrating state-of-the-art uniformity, fidelity and measurement statistics of spin ...
- The trouble with my listening room: speakers weren't made for iton May 1, 2024 at 11:30 pm
But it is where we have the biggest TV, with audio which varies somewhat according to incoming review equipment but is usually based on an AV-equipped Classé stereo amplifier and a pair of decade-old ...
- Remnant 2: Thorn Long Gun Explosive Buildson May 1, 2024 at 2:25 pm
The new Thorn Long Gun found in Remnant 2's Forgotten Kingdom DLC is a beast of a weapon. Let's look at some builds that use it the best.
- 12 things to do this weekend, from art openings to a community bike rideon May 1, 2024 at 9:22 am
The season of festivals, markets, outdoor recreation and spring shows is underway. Here's what to do this weekend in Pittsburgh.
- Organic electrochemical transistors: Scientists solve chemical mystery at the interface of biology and technologyon April 30, 2024 at 11:22 am
Researchers who want to bridge the divide between biology and technology spend a lot of time thinking about translating between the two different "languages" of those realms.
- Samsung to Introduce 3rd Generation Gate-All-Around 2nm Transistors in 2025on April 30, 2024 at 10:01 am
The company is already the industry leader when it comes to gate-all-around transistors, but so far it's yet to really make a dent in TSMC's market share.
- KittycasterFX’s Mohair Fuzzstortion is a reimagined Muff with a game-changing treble booster twiston April 30, 2024 at 8:38 am
Gee is not a fan of the four-transistor Muff-style circuit. “They’re very compressed,” he says. “Your picking attack is blunted off and it certainly doesn’t clean up from the volume knob of the guitar ...
via Bing News